Ever wonder how the body knows to perform certain functions? There are different forms of signals that pass between tissues in the body. These signals are recognized by proteins embedded in cell membranes so that they can pass the information to the inside of the cell and begin the required function.
The largest family of these protein receptors in the human body are called G-protein-coupled receptors (GPCRs). They can recognize signaling molecules such as light photons, peptides, lipids, sugars, and other proteins to detect what is going on in the body and relay the information to the inside of the cell. To do this, they interact with a number of internal cell factors known as G-proteins. When activated by external signals, GPCRs use the energy generated by the conversion of GTP to GDP to bind to their G-proteins and activate a cellular cascade of functions.
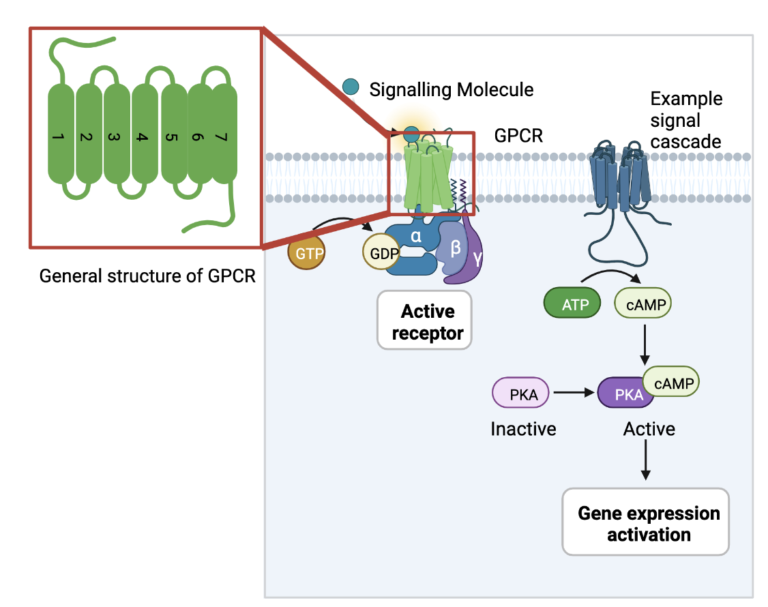
GPCRs are responsible for the regulation of many bodily functions, including growth and responses to hormone changes. When something goes wrong in this process, it can lead to downstream effects and produce diseases such as Alzheimer’s, hypo- and hyperthyroidism, fertility disorders, and even cancer. GPCRs serve as targets for drugs to stop harmful signals or resume healthy signaling. In order to design these drugs, the structure of the GPCR proteins must be studied to determine where the drug will bind and what might be causing the disorder in question. The following article focuses on new ways that scientists are using to study the structure of GPCRs.
Most GPCRs share common features that can be applied to different subtypes within the family. They are membrane proteins, meaning they are embedded in the cell membrane, and they consist of 7 transmembrane helices that move and change to receive and transmit signals. The study of GPCR structures in the interest of drug design has used 2 major strategies: x-ray crystallography and cryo-EM. These techniques are often employed in the field of protein structural biology, as proteins are too small to be seen under a normal light microscope. X-ray crystallography involves the generation of protein crystals, or protein molecules arranged in a lattice that solidifies out of solution and forms a regular, rigid structure. These protein crystals are then placed in front of a beam of x-rays, and they way that the x-rays diffract provides information for the placement of atoms of the protein.
The advantage of x-ray crystallography is that it can be applied to most any protein of any size and shape, even membrane proteins as in the case of GPCRs. Because GPCRs undergo a lot of changes as they receive and transmit signals, they move around quite a bit and require stabilization to form these regular crystal lattices. Thus, scientists have employed fusion proteins that bind to GPCRs and stabilize them into one rigid structure for study.
This is a very simplified explanation of this technique, for more thorough information I recommend this resource: Crystallography Made Crystal Clear (https://www.sciencedirect.com/book/9780125870733/crystallography-made-crystal-clear).
This is a very simplified explanation of this technique, for more thorough information I recommend this resource: Getting Started in Cryo-EM https://cryo-em-course.caltech.edu/ .
Another method of studying protein structures that has undergone recent advancement is cryogenic electron microscopy (cryo-EM). Instead of needing to form protein crystals, GPCR proteins are applied to a thin, circular carbon grid with small holes and then submerged in liquid ethane at -180oC to freeze it into a transparent (vitreous) layer of ice. This grid with vitreous ice and frozen GPCR protein particles is then placed in front of a stream of electrons, which pass through the ice but not the protein particles themselves, producing a 2D image of the proteins in ice. These 2D images can then be combined to view the full 3D model of the protein.
The advantage of cryo-EM is that it is much easier to prepare protein samples than for x-ray crystallography. However, since this technique is relatively new, scientists haven’t explored ways of keeping the protein from moving around too much. The more stable the GPCR protein structure is, the more 2D images can be collected, and the higher resolution the resulting 3D structure will be.
Remember how fusion proteins were being used in x-ray crystallography to stabilize the moving structure of GPCRs? This paper aims to illustrate how fusion proteins can also be employed to study GPCRs by cryo-EM. The authors of this paper used two different GPCRs as models for their technique because their full structures had already been determined: adenosine A2A receptor (A2AR) and smoothened receptor (SMO). This way, they could compare their results with fusion proteins and cryo-EM to previous results with fusion proteins and x-ray crystallography, and use what they learned to study new GPCRs.
The research conducted in this paper resulted in two successful structures from cryo-EM with some differences from x-ray crystallography techniques. The structure of A2AR was determined with a fusion protein called BRIL inserted and interacting with two of its seven transmembrane domains, stabilizing its structure but failing to provide high resolution images of the protein. Thus, researchers also used an antibody that recognized and bound to the BRIL fusion protein, making it even bigger and more obvious to choose particles and combine 2D images into a 3D structure. The structure of SMO could not be determined by the same technique because BRIL only bound to one transmembrane helix and did not adequately stabilize the structure. The authors used another fusion protein called PGS to stabilize the GPCR SMO. A contorted loop in the structure of the PGS fusion protein allowed it to interact with the SMO GPCR protein and stabilize it for cryo-EM data collection. Given these two similar strategies, the authors concluded that the use of fusion proteins to stabilize GPCRs is applicable to cryo-EM, but that each GPCR must be examined to determine the proper fusion protein to use.
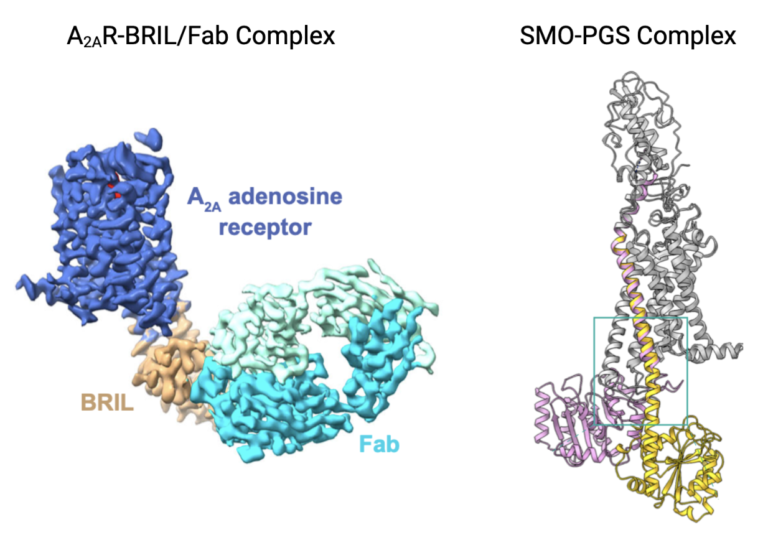
How proteins are important
GPCRs are a large and diverse category of transmembrane receptors that allow the cells to receive and respond to signals from the body. Their mutations and level of activity are associated with different diseases, and they are important drug targets to fight these diseases. Understanding how these GPCRs look structurally is necessary to understanding how they function, and how to control this function. Researching better ways to study these proteins is important for the development of medicine, and applications to other transmembrane proteins.
Fusion proteins are small proteins designed to insert and bind to a larger protein or construct to stabilize the structure. Their design is crucial for the stabilization of the larger protein of interest, for not every fusion protein will stabilize the target enough to get good images from cryo-EM. Understanding how fusion proteins interact effectively with the target protein of interest, like GPCRs, helps in the design of future constructs to collect better data for structural analysis.